IRG 2: ACTIVATED ARCHITECTURED MATERIALS
We design and build shape-morphing hybrid materials with programmable and spatiotemporally self-regulating transport properties.
The ability to create active materials with distributed microscopic elements that convert energy into local mechanical work would fundamentally alter existing approaches to materials design.
Biology offers many examples of these materials, where they impart autonomy to living systems. Inspired by nature, we will design and build hybrid inorganic-biological materials that sense and interact with the environment to produce an “artificial” skin and active ink-jet drops to enable reconfigurable printing.
Developing ‘activated’ materials
Our group will develop these technologies on a platform that consists of “activated” materials. By this we mean materials that operate inherently out of equilibrium because they are comprised of either (1) chemically active components, such as molecular motors, (2) building blocks driven by suitably tailored external fields, or (3) both, acting in tandem.
Attempts to create such materials have to date been restricted to living systems that contain mechanoenzymes or limited classes of model systems (for example, colloidal swimmers and chiral fluids).
Our team seeks to capitalize on new opportunities that arise when approaches from active matter are combined with recent advances in the synthesis of inorganic materials that exploit their unique mechanical, optical, thermal, and electrical properties.
The resulting hybrid structures, which we refer to as activated architectured materials, will harness the feedback between activation, local architecture, and transport.
That feedback can proceed along two distinct routes. In route 1, activation modifies transport properties such as the viscosity of an active fluid, leading to additional active stresses that can change material architecture (for example, changing the shape of a droplet). In route 2, activation directly impacts the structure of a material—for example, the conformation of an activated sheet—which in turn leads to modification of its thermal transport properties.
Exploring routes of activation
We have organized our research to take advantage of exciting opportunities in the field and to build on our strengths.
Focus area 1 explores route 1 using activated fluids composed of self-spinning colloids and nanoparticles as model systems. Here, modulations in the external drive provide a means for spatiotemporal control of active stresses, paving the way for self-printing ink-jet drops. We call these fluids metafluids, analogous to metamaterials, because their properties are engineered by a suitably controlled external drive.
Focus area 2 explores route 2 using activated sheets composed of hybrid passive-inorganic and active-biological components. Thin sheets offer a particularly promising platform because of the soft bending modes that can be activated by biomolecular motors. Here, we will employ shape-shifting materials powered by active stresses to control optical and thermal conductivity.
Focus area 3 brings together elements of the first two and considers composite structures with multiple independent sources of activation. For instance, we will construct “artificial skin” by combining epithelial cells—which are themselves chemically active—with soft polymer electronics that provide an additional source of activation. Here, we will attempt to achieve the level of integrated biomechanical functionalities that are typically associated with living systems.
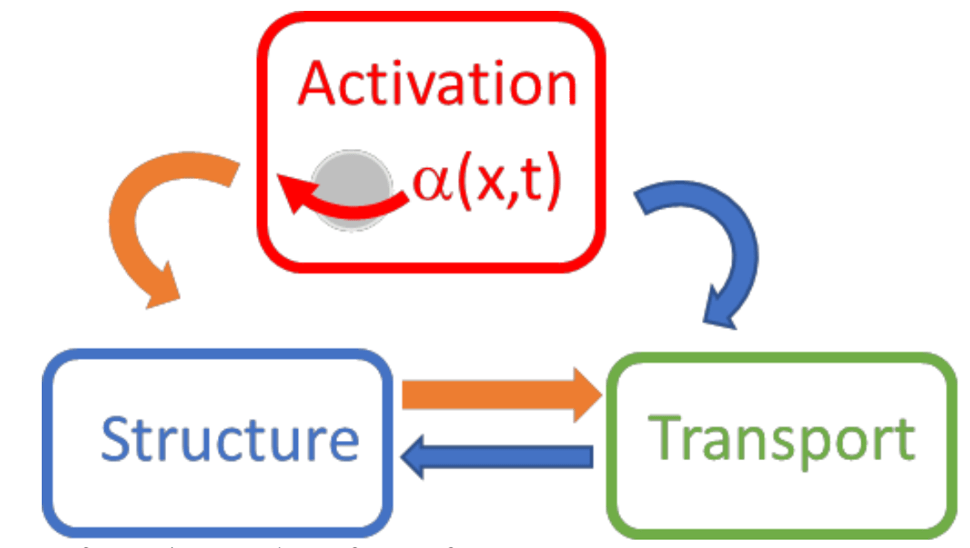
Material components (gray circles) are activated with spatial and/or time control (α(x,t)) to drive local motion and force (red arrow). This local activation controls material response through two routes, as described in the text.
Senior faculty
- Juan de Pablo
- Margaret Gardel
- William Irvine
- Ka Yee Lee
- Jiwoong Park (coordinator)
- Dmitri Talapin
- Suri Vaikuntanathan
- Vincenzo Vitelli (coordinator)
- Thomas Witten